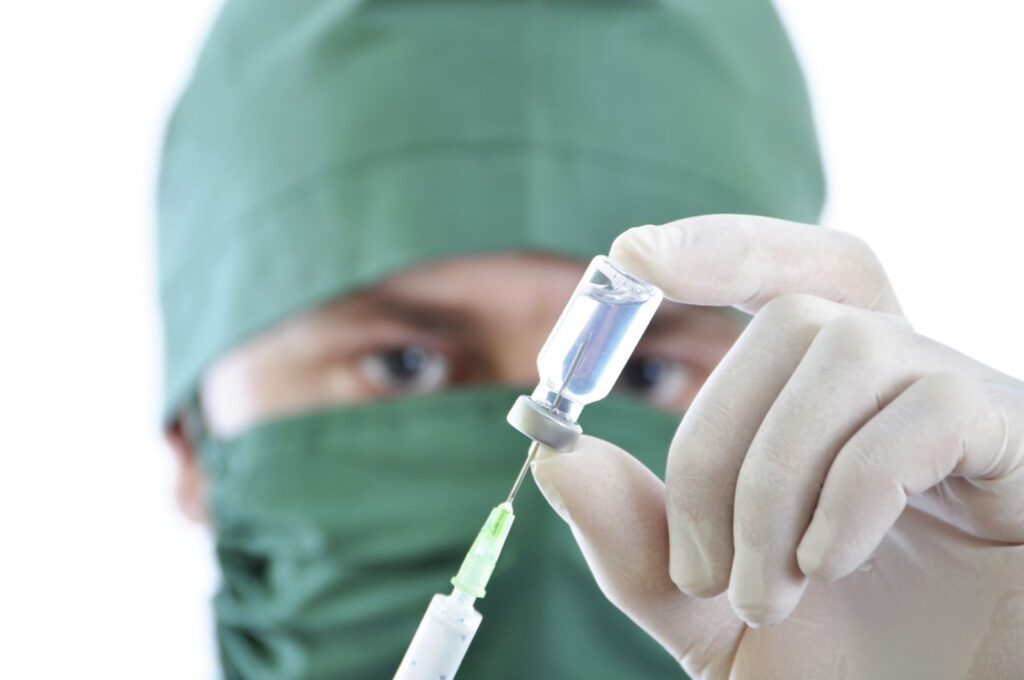
Different tissues are affected by anesthetics in varying ways, largely depending on blood flow and the tissue’s ability to store the anesthetic. The time to reach equilibrium with the anesthetic gas’s partial pressure is inversely related to tissue blood flow (greater flow equals shorter time) and directly related to the tissue’s storage capacity (greater capacity equals longer time). Storage capacity itself is proportional to the tissue volume and the gas’s solubility in that tissue. Four main tissue compartments affect the anesthetic uptake time:
- Vessel-rich group (brain, heart, liver, kidney, and endocrine glands): These highly perfused tissues quickly reach equilibrium with the blood’s anesthetic partial pressure.
- Skeletal muscles: These are moderately perfused with a high storage capacity, delaying the time to reach equilibrium.
- Fat: Although fat is poorly perfused, it has a large storage capacity for lipophilic volatile anesthetics, significantly prolonging equilibrium time.
- Vessel-poor group (bone, ligaments, and cartilage): These tissues are very poorly perfused and have a low capacity for anesthetic gas, thus minimally affecting the anesthetic distribution time.
Washout: When inhalation of an anesthetic gas stops, the body releases the stored anesthetic back to the alveoli. Factors affecting the uptake and equilibrium of the anesthetic also influence its exhalation. Thus, nitrous oxide is exhaled faster than isoflurane.
Mechanism of Action: No specific receptor has been identified as responsible for general anesthesia. The variety of chemically different compounds that induce unconsciousness suggests multiple mechanisms. At clinically effective concentrations, general anesthetics enhance the sensitivity of GABA receptors to the inhibitory neurotransmitter GABA, increasing chloride ion influx and hyperpolarizing neurons, thereby reducing CNS activity. Nitrous oxide and ketamine, however, act on NMDA receptors rather than GABA receptors. Other receptors affected by volatile anesthetics include inhibitory glycine receptors in spinal motor neurons and nicotinic receptors involved in excitatory postsynaptic currents. The precise mechanisms remain unclear.
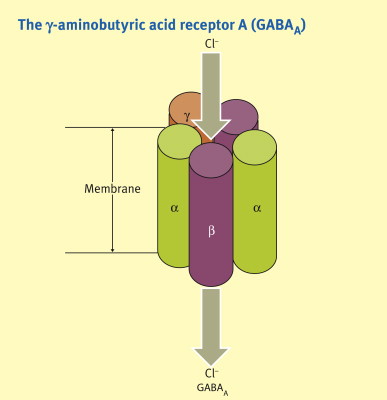
Isoflurane: Isoflurane, a halogenated gas, causes dose-dependent hypotension primarily by relaxing systemic blood vessels. It can be treated with a direct-acting vasoconstrictor like phenylephrine. Isoflurane undergoes minimal metabolism, making it non-toxic to the liver and kidneys. Due to its pungent odor, which can cause respiratory reflexes such as breath-holding and coughing, it is not suitable for inhalation induction. Its higher blood solubility means it takes longer to reach equilibrium, making it less ideal for short procedures, but its low cost is advantageous for longer surgeries.
Desflurane: Desflurane offers rapid onset and recovery due to its low blood solubility, making it popular for short procedures. It requires a special heated vaporizer due to its low volatility. Similar to isoflurane, desflurane decreases vascular resistance and perfuses major tissues well. It also causes respiratory irritation, so it is not used for inhalation induction. Desflurane has minimal degradation and rare tissue toxicity, though its higher cost can be a drawback.
Sevoflurane: Sevoflurane has low pungency and respiratory irritation, making it suitable for inhalation induction, especially in pediatric patients. It offers rapid onset and recovery due to low blood solubility and has low hepatotoxic potential. However, compounds formed during reactions in the anesthesia circuit may be nephrotoxic with prolonged low fresh gas flow.
Nitrous Oxide: Nitrous oxide, often used in combination with oxygen for moderate sedation, particularly in dentistry, maintains respiratory and muscular function. It can be used with other inhalation agents to enhance general anesthesia, reducing the required concentration of the other agent and mitigating its side effects. Nitrous oxide’s poor solubility allows rapid movement in and out of the body, which can cause issues in closed body compartments. It can lead to “diffusion hypoxia” during recovery, which can be prevented with high concentrations of inspired oxygen.
Malignant Hyperthermia (MH): In rare cases, exposure to halogenated anesthetics or succinylcholine can trigger MH, a life-threatening condition characterized by uncontrolled skeletal muscle metabolism. This overwhelms the body’s oxygen supply, CO2 removal, and temperature regulation, potentially causing circulatory collapse and death if untreated. MH is linked to a defect In muscle excitation-contraction coupling and is often inherited as an autosomal dominant disorder. Immediate treatment with dantrolene, which inhibits calcium release from the sarcoplasmic reticulum, is crucial, along with cooling and supportive measures. The use of dantrolene and avoiding triggering agents have significantly reduced MH mortality.
Conclusion
In conclusion, the distribution and effects of inhalation anesthetics in the body are influenced by various factors such as tissue perfusion, storage capacity, and the specific mechanisms of action on neurotransmitter receptors. Tissues with high blood flow, like the brain and heart, reach equilibrium quickly with anesthetic gases, while those with high storage capacity, such as fat, prolong the time required to achieve steady state. Understanding these dynamics is crucial for optimizing anesthesia delivery and managing patient outcomes.
Furthermore, the mechanisms by which anesthetics induce general anesthesia are diverse, involving modulation of GABA receptors, NMDA receptors, glycine receptors, and others. This complexity underscores the challenge in identifying a singular receptor responsible for anesthesia induction.
Each inhalation anesthetic, whether isoflurane, desflurane, sevoflurane, or nitrous oxide, offers distinct pharmacokinetic and pharmacodynamic properties that cater to specific clinical needs. From rapid onset and recovery to minimal organ toxicity, these characteristics guide their selection for different surgical scenarios.
Lastly, awareness of complications such as malignant hyperthermia highlights the importance of vigilance and preparedness in anesthesia practice. Prompt recognition and treatment with agents like dantrolene can significantly mitigate risks associated with anesthesia-induced hypermetabolic states.
In clinical practice, balancing these factors ensures safe and effective anesthesia delivery, tailored to individual patient needs and procedural requirements.