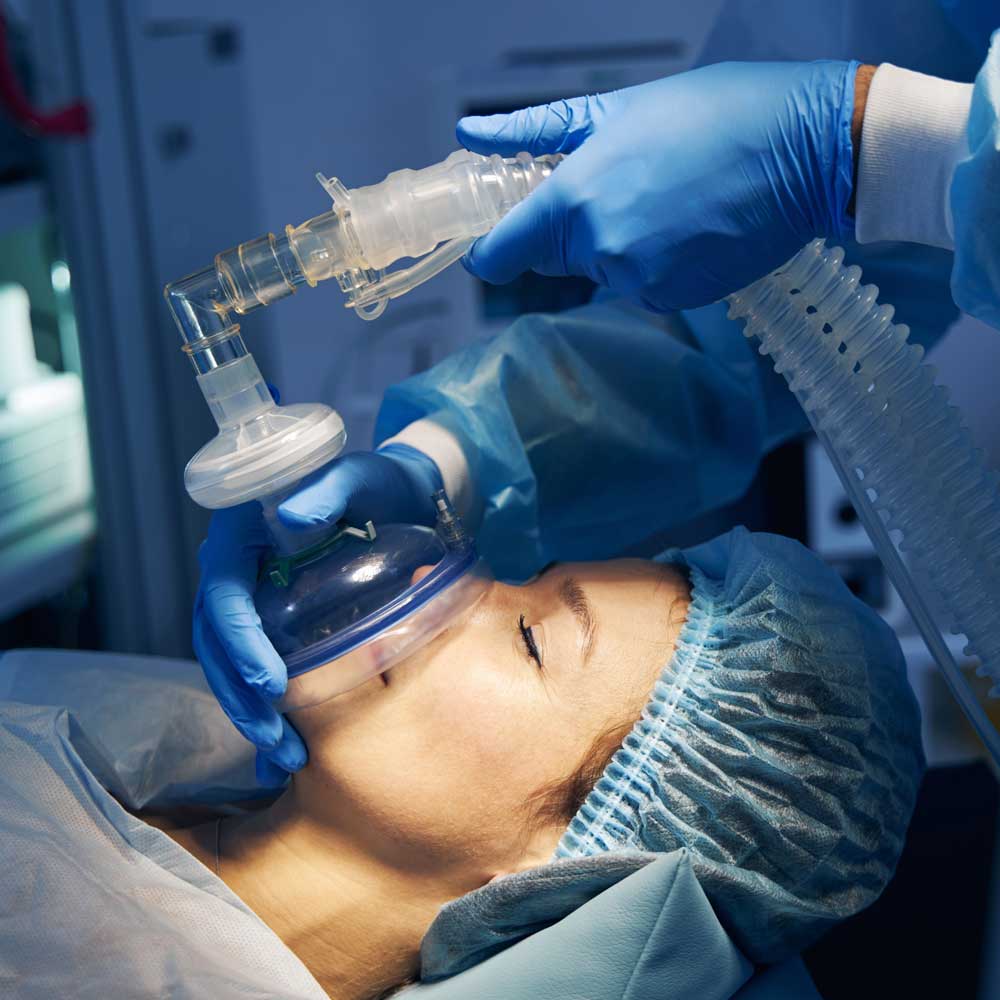
Inhaled gases are primarily used for maintaining anesthesia after an initial IV drug administration. The depth of anesthesia can be quickly adjusted by altering the concentration of the inhaled gas. These agents have steep dose-response curves and narrow therapeutic indices, meaning that the margin between achieving anesthesia and causing cardiopulmonary collapse is slim. There are no antagonists available for these gases. To reduce waste, these gases are delivered through a recirculation system that includes absorbents to remove carbon dioxide, allowing the gases to be rebreathed. Recently, there has been growing concern about the environmental impact of these potent greenhouse gases, which are typically released from hospital rooftops after procedures.
A. Common Features of Inhalation Anesthetics
Modern inhalation anesthetics, such as nitrous oxide and halogenated hydrocarbons, are nonflammable and nonexplosive. They decrease cerebrovascular resistance, leading to increased brain perfusion, cause bronchodilation, and reduce both respiratory drive and hypoxic pulmonary vasoconstriction. The movement of these gases from the lungs to different body compartments depends on their solubility in blood and tissues, as well as blood flow. Several factors influence the induction and recovery of anesthesia.
B. Potency
Potency is quantitatively defined by the minimum alveolar concentration (MAC), the end-tidal concentration of inhaled anesthetic required to eliminate movement in 50% of patients exposed to a painful stimulus. MAC is essentially the median effective dose (ED) of the anesthetic, expressed as the percentage of gas in a mixture needed to achieve that effect. Potent anesthetics like isoflurane have a small MAC value, while less potent agents like nitrous oxide have a large MAC value.
Therefore, the inverse of MAC is an index of potency. Nitrous oxide alone cannot produce general anesthesia because its MAC value cannot be achieved when mixed with a survivable oxygen percentage. More lipid-soluble anesthetics require lower concentrations to produce anesthesia, resulting in higher potency. Factors that increase MAC include hyperthermia, drugs that elevate CNS catecholamines, and chronic alcohol use. Conversely, factors that decrease MAC include advanced age, hypothermia, pregnancy, sepsis, acute intoxication, concurrent IV anesthetics, and use of β-adrenergic receptor agonists like clonidine and dexmedetomidine.
C. Uptake and Distribution of Inhalation Anesthetics
The main goal of inhalation anesthesia is to achieve a constant and optimal brain partial pressure (P) of the inhaled anesthetic. The movement of the anesthetic gas from the lungs to the brain and other body compartments is determined by partial pressure gradients. At equilibrium, partial pressures in various compartments are equal. The time required to reach this steady state is influenced by several factors.
Alveolar Transfer: The replacement of normal lung gases with the inhaled anesthetic mixture is proportional to the lung’s functional residual capacity (volume of gas remaining in the lungs at the end of a normal expiration) and inversely proportional to the respiratory rate. This process is independent of the gas’s physical properties. As the partial pressure in the lungs builds, the transfer of anesthetic gas from the lungs begins.
Anesthetic Uptake: This involves the removal of the gas to peripheral tissues other than the brain. Uptake is a product of the gas’s solubility in blood, cardiac output (CO), and the gradient between alveolar and blood anesthetic partial pressures.
Solubility in Blood: Determined by the blood-gas partition coefficient, which is the ratio of the concentration of anesthetic in the liquid (blood) phase to the gas phase when the anesthetic is at equilibrium between the two phases. Blood acts as a pharmacologically inactive reservoir for inhaled anesthetics.
Anesthetics with low blood solubility, like nitrous oxide, reach equilibrium between the inspired anesthetic and arterial blood quickly because little anesthetic dissolves in the blood. In contrast, highly soluble anesthetics like isoflurane require more gas and longer periods to raise blood partial pressure, resulting in longer induction and recovery times and slower changes in anesthesia depth.
Cardiac Output: There is an inverse correlation between CO and induction time for inhaled anesthetics. With low CO, a larger concentration of gas dissolves in the blood, providing a longer exposure time for the drug to diffuse into neuronal tissue. High CO transports the drug to the brain quickly but at a lower concentration, slowing induction.
Alveolar-to-Venous Partial Pressure Gradient: This gradient results from tissue uptake from arterial delivery. Tissue uptake depends on tissue blood flow, blood-to-tissue partial pressure difference, and blood-to-tissue solubility. As venous blood returns to the lungs with little or no dissolved anesthetic gas, the gradient causes gas to move from the alveoli into the blood. A high gradient indicates high peripheral tissue uptake, prolonging induction time. Over time, as the partial pressure of gas in venous blood approaches the inspired mixture, uptake from the lung ceases.
Conclusion
- Inhaled anesthetics play a crucial role in maintaining anesthesia after initial IV administration, allowing for rapid adjustments in anesthetic depth. These agents have a narrow therapeutic index, which necessitates careful control to avoid adverse effects such as cardiopulmonary collapse. Delivered via recirculation systems to minimize waste, these anesthetics are potent greenhouse gases, raising environmental concerns.
- Key features of inhaled anesthetics include their nonflammable nature and their impact on the body, such as increasing brain perfusion and causing bronchodilation while decreasing respiratory drive. The potency of these anesthetics is measured by the minimum alveolar concentration (MAC), with more lipid-soluble agents being more potent.
- The uptake and distribution of inhaled anesthetics are influenced by factors such as solubility in blood, cardiac output, and alveolar-to-venous partial pressure gradients. These factors affect the induction and recovery times and the ability to change the depth of anesthesia. Anesthetics with low blood solubility achieve rapid equilibrium and thus faster induction and recovery, whereas those with high blood solubility require more time.